CERN PRESS RELEASE: 4th July 2012
http://press.web.cern.ch/press/PressReleases/Releases2012/PR17.12E.html
“We observe in our data clear signs of a new particle, at the level of 5 sigma, in the mass region around 126 GeV. The outstanding performance of the LHC and ATLAS and the huge efforts of many people have brought us to this exciting stage,” said ATLAS experiment spokesperson Fabiola Gianotti, “but a little more time is needed to prepare these results for publication.”
"The results are preliminary but the 5 sigma signal at around 125 GeV we’re seeing is dramatic. This is indeed a new particle. We know it must be a boson and it’s the heaviest boson ever found,” said CMS experiment spokesperson Joe Incandela. “The implications are very significant and it is precisely for this reason that we must be extremely diligent in all of our studies and cross-checks."
“It’s hard not to get excited by these results,” said CERN Research Director Sergio Bertolucci. “ We stated last year that in 2012 we would either find a new Higgs-like particle or exclude the existence of the Standard Model Higgs. With all the necessary caution, it looks to me that we are at a branching point: the observation of this new particle indicates the path for the future towards a more detailed understanding of what we’re seeing in the data.”
The results presented today are labelled preliminary. They are based on data collected in 2011 and 2012, with the 2012 data still under analysis. Publication of the analyses shown today is expected around the end of July. A more complete picture of today’s observations will emerge later this year after the LHC provides the experiments with more data.
The next step will be to determine the precise nature of the particle and its significance for our understanding of the universe. Are its properties as expected for the long-sought Higgs boson, the final missing ingredient in the Standard Model of particle physics? Or is it something more exotic? The Standard Model describes the fundamental particles from which we, and every visible thing in the universe, are made, and the forces acting between them. All the matter that we can see, however, appears to be no more than about 4% of the total. A more exotic version of the Higgs particle could be a bridge to understanding the 96% of the universe that remains obscure.
“We have reached a milestone in our understanding of nature,” said CERN Director General Rolf Heuer. “The discovery of a particle consistent with the Higgs boson opens the way to more detailed studies, requiring larger statistics, which will pin down the new particle’s properties, and is likely to shed light on other mysteries of our universe.”
Positive identification of the new particle’s characteristics will take considerable time and data. But whatever form the Higgs particle takes, our knowledge of the fundamental structure of matter is about to take a major step forward.
SCIENCE DEVELOPMENT NETWORK REFLECTS ON UTILITY OF HIGGS BOSON FOR COMMON MAN
Big scientific discoveries, such as the God particle, can have great Earthly potential that must be shared by the world's poor.
The announcement from CERN that we now have compelling evidence for the Higgs boson — the elementary particle that, according to theory, allows other particles to obtain mass — is widely acknowledged as a significant contribution to our understanding of the universe.
But thoughts have quickly turned to the implications of this momentous discovery — and about the value of the investment of public money, particularly in an age of austerity.
What might it offer our quality of life here on Earth? Will this so-called God particle provide the benevolence we associate with a deity by contributing to health or reducing drudgery?
The reality is that it is much too early for us to know what we might be able to achieve. Scientists have compared it to getting around a corner of a street or finding a door to a room no-one has entered before. This may just be the start of a new chain of insights to keep generations of scientists busy.
From this perspective, querying the poverty-alleviation credentials of the God particle seems churlish. But applications such as the transistor came soon from the field of quantum mechanics, so the important question still remains: how do we maximise opportunities presented by discoveries like this to respond to the challenges facing communities in developing countries in the medium- to long-term?
Competing research agendas
Experience suggests that the research and development (R&D) agendas that stem from important discoveries do not favour the priorities of the poor.
Typically, developing countries end up participating in innovations only in as far as the market needs them. Our latest Spotlight explores the barriers that local innovators in these countries face when trying to get into the international high-tech R&D market. And there are barriers too for innovators in these countries working in more conventional R&D systems such as science institutes.
There is a mix of factors that undermine the ethical and intelligent procurement of research. They include geopolitical agendas on security, as defence budgets command many research resources; international competition for research resources, which fuels the brain drain; and of course, the profit motive, which drives most capital investment, and which has spawned an army of corporate lobbyists looking for state funding.
Military agendas dominated soon after the discovery of nuclear fission, for example; and even developing countries with the infrastructure to exploit nuclear power as a source of clean energy have instead used it to achieve military ambitions.
Philanthropy and citizens united
There is hope. Take malaria, which has been a scourge to millions across the developing world for generations; even now a child is believed to die every 45 seconds in Africa from the disease. Researchers and activists have been complaining about the lack of sustained commitment to tackling the disease.
But in the last seven years, we have seen a significant growth in funding for vaccine research. Last year, Bill Gates told an informal gathering in London that there are several under-resourced diseases in the developing world for philanthropists to choose from.
Gates' own foundation, which had made malaria an early priority, and similar charitable initiatives in emerging economies and online (Kiva, a microfinance website, has interesting potential), provide important momentum for change.
Citizen philanthropy has more than doubled in terms of funding for international development over the past decade, presenting new opportunities for the international community to set the agenda on research needs. It is helping to resist — at least for now — the gravitational pull on research resources from the military and corporate lobbying.
Another area of opportunity lies in re-invigorating public-private partnerships. Many campaigners working on malaria, for instance, recognise the importance of working with business models for sustained delivery of interventions such as bednets; intellectual property rights for vaccine research; and health systems research to improve healthcare.
It has done this by investing in promising businesses and working with local fund managers to maximise returns, taking into account environmental and social impact. The institute has worked in a wide range of sectors, from pharmaceuticals to information technology to real estate, and often with the complex stakeholder communities that we might associate with campaigners working on malaria, for instance.
As a venture capital mechanism, the CDC provides useful experience of reconciling a development agenda with capitalist instincts and matching funding to demand. Surely the time has come to think about how we might apply such experience to research.
And this leads us to the earliest implication of the Higgs boson discovery. It is a lesson in moral imperatives. This God particle reminds us of our shared humanity — that getting research insights applied to alleviating poverty matters, and is the least of what we are capable of.
Nick Ishmael Perkins
Director, SciDev.Net
--------------
NEW YORK TIMES OPED challenges excitement out of HIGGS BOSON
http://press.web.cern.ch/press/PressReleases/Releases2012/PR17.12E.html
CERN experiments observe particle consistent with long-sought Higgs boson
Geneva, 4 July 2012. At a seminar held at CERN1 today as a curtain raiser to the year’s major particle physics conference, ICHEP2012 in Melbourne, the ATLAS and CMS experiments presented their latest preliminary results in the search for the long sought Higgs particle. Both experiments observe a new particle in the mass region around 125-126 GeV.“We observe in our data clear signs of a new particle, at the level of 5 sigma, in the mass region around 126 GeV. The outstanding performance of the LHC and ATLAS and the huge efforts of many people have brought us to this exciting stage,” said ATLAS experiment spokesperson Fabiola Gianotti, “but a little more time is needed to prepare these results for publication.”
"The results are preliminary but the 5 sigma signal at around 125 GeV we’re seeing is dramatic. This is indeed a new particle. We know it must be a boson and it’s the heaviest boson ever found,” said CMS experiment spokesperson Joe Incandela. “The implications are very significant and it is precisely for this reason that we must be extremely diligent in all of our studies and cross-checks."
“It’s hard not to get excited by these results,” said CERN Research Director Sergio Bertolucci. “ We stated last year that in 2012 we would either find a new Higgs-like particle or exclude the existence of the Standard Model Higgs. With all the necessary caution, it looks to me that we are at a branching point: the observation of this new particle indicates the path for the future towards a more detailed understanding of what we’re seeing in the data.”
The results presented today are labelled preliminary. They are based on data collected in 2011 and 2012, with the 2012 data still under analysis. Publication of the analyses shown today is expected around the end of July. A more complete picture of today’s observations will emerge later this year after the LHC provides the experiments with more data.
The next step will be to determine the precise nature of the particle and its significance for our understanding of the universe. Are its properties as expected for the long-sought Higgs boson, the final missing ingredient in the Standard Model of particle physics? Or is it something more exotic? The Standard Model describes the fundamental particles from which we, and every visible thing in the universe, are made, and the forces acting between them. All the matter that we can see, however, appears to be no more than about 4% of the total. A more exotic version of the Higgs particle could be a bridge to understanding the 96% of the universe that remains obscure.
“We have reached a milestone in our understanding of nature,” said CERN Director General Rolf Heuer. “The discovery of a particle consistent with the Higgs boson opens the way to more detailed studies, requiring larger statistics, which will pin down the new particle’s properties, and is likely to shed light on other mysteries of our universe.”
Positive identification of the new particle’s characteristics will take considerable time and data. But whatever form the Higgs particle takes, our knowledge of the fundamental structure of matter is about to take a major step forward.
SCIENCE DEVELOPMENT NETWORK REFLECTS ON UTILITY OF HIGGS BOSON FOR COMMON MAN
From Higgs to humanity: discoveries for the common good
13 July 2012 |
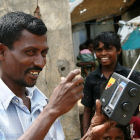
Transistor radios have been developed from discoveries in the field of quantum mechanics
Flickr/Internews network
Big scientific discoveries, such as the God particle, can have great Earthly potential that must be shared by the world's poor.
The announcement from CERN that we now have compelling evidence for the Higgs boson — the elementary particle that, according to theory, allows other particles to obtain mass — is widely acknowledged as a significant contribution to our understanding of the universe.
But thoughts have quickly turned to the implications of this momentous discovery — and about the value of the investment of public money, particularly in an age of austerity.
What might it offer our quality of life here on Earth? Will this so-called God particle provide the benevolence we associate with a deity by contributing to health or reducing drudgery?
The reality is that it is much too early for us to know what we might be able to achieve. Scientists have compared it to getting around a corner of a street or finding a door to a room no-one has entered before. This may just be the start of a new chain of insights to keep generations of scientists busy.
From this perspective, querying the poverty-alleviation credentials of the God particle seems churlish. But applications such as the transistor came soon from the field of quantum mechanics, so the important question still remains: how do we maximise opportunities presented by discoveries like this to respond to the challenges facing communities in developing countries in the medium- to long-term?
Competing research agendas
Experience suggests that the research and development (R&D) agendas that stem from important discoveries do not favour the priorities of the poor.
Typically, developing countries end up participating in innovations only in as far as the market needs them. Our latest Spotlight explores the barriers that local innovators in these countries face when trying to get into the international high-tech R&D market. And there are barriers too for innovators in these countries working in more conventional R&D systems such as science institutes.
There is a mix of factors that undermine the ethical and intelligent procurement of research. They include geopolitical agendas on security, as defence budgets command many research resources; international competition for research resources, which fuels the brain drain; and of course, the profit motive, which drives most capital investment, and which has spawned an army of corporate lobbyists looking for state funding.
Military agendas dominated soon after the discovery of nuclear fission, for example; and even developing countries with the infrastructure to exploit nuclear power as a source of clean energy have instead used it to achieve military ambitions.
Philanthropy and citizens united
There is hope. Take malaria, which has been a scourge to millions across the developing world for generations; even now a child is believed to die every 45 seconds in Africa from the disease. Researchers and activists have been complaining about the lack of sustained commitment to tackling the disease.
But in the last seven years, we have seen a significant growth in funding for vaccine research. Last year, Bill Gates told an informal gathering in London that there are several under-resourced diseases in the developing world for philanthropists to choose from.
Gates' own foundation, which had made malaria an early priority, and similar charitable initiatives in emerging economies and online (Kiva, a microfinance website, has interesting potential), provide important momentum for change.
Citizen philanthropy has more than doubled in terms of funding for international development over the past decade, presenting new opportunities for the international community to set the agenda on research needs. It is helping to resist — at least for now — the gravitational pull on research resources from the military and corporate lobbying.
Another area of opportunity lies in re-invigorating public-private partnerships. Many campaigners working on malaria, for instance, recognise the importance of working with business models for sustained delivery of interventions such as bednets; intellectual property rights for vaccine research; and health systems research to improve healthcare.
Model from the United Kingdom
The UK Department for International Development (DFID) owns the world's oldest development finance institute, the CDC, which has returned a profit of £1.8 billion (US$2.8 billion), all re-invested in the institute, since 2004.
The UK Department for International Development (DFID) owns the world's oldest development finance institute, the CDC, which has returned a profit of £1.8 billion (US$2.8 billion), all re-invested in the institute, since 2004.
It has done this by investing in promising businesses and working with local fund managers to maximise returns, taking into account environmental and social impact. The institute has worked in a wide range of sectors, from pharmaceuticals to information technology to real estate, and often with the complex stakeholder communities that we might associate with campaigners working on malaria, for instance.
As a venture capital mechanism, the CDC provides useful experience of reconciling a development agenda with capitalist instincts and matching funding to demand. Surely the time has come to think about how we might apply such experience to research.
And this leads us to the earliest implication of the Higgs boson discovery. It is a lesson in moral imperatives. This God particle reminds us of our shared humanity — that getting research insights applied to alleviating poverty matters, and is the least of what we are capable of.
Nick Ishmael Perkins
Director, SciDev.Net
NEW YORK TIMES OPED challenges excitement out of HIGGS BOSON
Op-Ed Contributor
Why the Higgs Boson Matters

Daniel Haskett
By STEVEN WEINBERG
Published: July 13, 2012

We have a well-tested theory of elementary particles and the forces that
they exert on each other, known as the Standard Model. A central
feature of the Standard Model is a symmetry between two of these forces:
the electromagnetic force, and the less familiar weak nuclear force,
which provides the first step in the chain of reactions that gives the
sun its energy.
The symmetry means that the particles carrying these forces enter into
the equations of the theory in essentially the same way. You could
interchange the photon, the particle of light that carries the
electromagnetic force, with some combination of the W and Z particles
that carry the weak nuclear force, and the equations would be unchanged.
If nothing intervened to break this symmetry, the W and Z, like the
photon, would have no mass. In fact, all other elementary particles
would also be massless. But of course, most elementary particles are not
massless. For instance, unlike the massless photon, the W and Z
particles have nearly 100 times the mass of a hydrogen atom.
Since the early 1960s it has been known that it is possible for
symmetries to be exact properties of the equations of a theory and yet
not respected by observable physical quantities, like the values of
particle masses. The consequences of such symmetry breaking were worked
out in 1964 by Robert Brout and François Englert; by Peter Higgs; and by
Gerald Guralnik, Carl Hagen and Tom Kibble, for a general class of
theories that contain force-carrying particles, like the photon.
In 1967-8 the late Abdus Salam and I independently used this mathematics
in formulating a specific theory, the modern unified theory of weak and
electromagnetic forces that became part of the Standard Model. This
theory predicted the masses of the W and Z particles, which were
verified when these particles were discovered at CERN in 1983-84.
But just what is it that breaks the electroweak symmetry and thereby gives elementary particles their masses?
Salam and I assumed that the culprit is what are called scalar fields,
which pervade all space. This is like what happens in a magnet: Even
though the equations describing iron atoms don’t distinguish one
direction in space from another, any magnetic field produced by the
atoms will point in just one way. The symmetry-breaking fields in the
Standard Model do not mark out directions in space — instead, they
distinguish the weak from the electromagnetic forces, and give
elementary particles their masses. Just as a magnetic field appears in
iron when it cools and solidifies, these scalar fields appeared as the
early universe expanded and cooled.
This is where the Higgs boson comes in. The illustrative models studied
in most of the papers on symmetry breaking from 1960 to 1964 had
introduced scalar fields to break the symmetries, and had typically
found that some of these fields would show up as massive particles,
bundles of the energy of the fields. Likewise, Salam and I in 1967-68
found that one of the four scalar fields we introduced to break the
electroweak symmetry would appear as a new kind of electrically neutral
unstable particle. This is the Higgs boson, which may now have been
discovered, verifying the Standard Model’s account of how the elementary
particles get their masses.
There seems no doubt that a new electrically neutral, unstable particle
had been discovered, but is it the Higgs boson? All of the properties of
the Higgs boson except its mass were predicted in the 1967-8
electroweak theory, and since the mass of the new particle has been
measured, we can now calculate the probabilities for the various ways
that it can decay. So far, only a few decay modes have been observed,
and though the new particle seems to decay like a Higgs boson, more must
be done to pin this down. Also, if the new particle is the Higgs boson,
it would have to be like a knuckleball in baseball; unlike all other
known elementary particles, it would have no spin. This too must be
tested.
These are the cautious words you would expect to hear from a prudent
physicist. But I have been waiting for the discovery of the Higgs boson
since 1967, and it’s hard for me now to doubt that it has been found.
So what? Even if the particle is the Higgs boson, it is not going to be
used to cure diseases or improve technology. This discovery simply fills
a gap in our understanding of the laws of nature that govern all
matter, and throws light on what was going on in the early universe.
It’s wonderful that many people do care about this sort of science, and
regard it as a credit to our civilization.
Of course not everyone feels this way, and even those who do have to ask
whether learning the laws of nature is worth the billions of dollars it
costs to build particle accelerators. This question is going to come up
again, since our present Standard Model is certainly not the end of the
story. It leaves out gravitation; it does not explain the particular
values of the masses of quarks and electrons and other particles; and
none of its particles can account for the “dark matter” that astronomers
tell us makes up five-sixths of the mass of the universe. You can count
on physicists to ask their governments for the facilities they need to
grapple with these problems.
A case can be made for this sort of spending, even to those who don’t
care about learning the laws of nature. Exploring the outer frontier of
our knowledge of nature is in one respect like war: It pushes modern
technology to its limits, often yielding new technology of great
practical importance.
For instance, the new particle was produced at CERN in collisions of
protons that occur at a rate of over a hundred million collisions per
second. To analyze the flood of data produced by all these collisions
requires real time computing of unmatched power. Also, before the
protons collide, they are accelerated to an energy over 3,000 times
larger than the energy contained in their own masses while they go many
times around a 27-kilometer circular tunnel. To keep them in their
tracks requires enormously strong superconducting magnets, cooled by the
world’s largest source of liquid helium. In previous work at CERN,
elementary particle physicists developed a method of sharing data that
has become the World Wide Web.
On a longer time scale, the advance of technology will reflect the
coherent picture of nature we are now assembling. At the end of the 19th
century physicists in England were exploring the properties of electric
currents passing through a near vacuum. Although this was pure science,
it led to our knowledge of the electron, without which a large part of
today’s technology would be impossible. If these physicists had limited
themselves to work of obvious practical importance, they would have been
studying the behavior of steam boilers.
Steven Weinberg is a professor in the physics and
astronomy departments at the University of Texas at Austin, and the
recipient of the Nobel Prize in Physics for his work on the unified
theory of weak and electromagnetic forces.